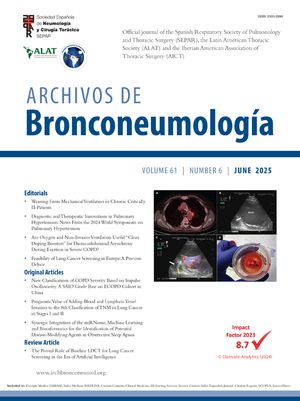
Lung cancer is one of the most common causes of cancer death in men and women worldwide. Various combinations of surgery, chemotherapy, radiation therapy and immunotherapy are currently used to treat lung cancer. However, the prognosis remains relatively poor due to the higher frequency of tumor mutational burden (TMB). Nuclear factor E2-related factor 2 (NFE2L2/NRF2) is often considered a primary regulator of the expression of antioxidant enzymes and detoxification proteins and is involved in cytoprotection. On the contrary, NRF2 is even known to induce metastasis and support tumor progression. Kelch-like ECH-associated protein 1 (KEAP1) plays an important role in negatively regulating NRF2 activity via CUL3-mediated ubiquitinylation and successive proteasomal degradation. Extensive research has shown that the genetic alterations of KEAP1/NFE2L2/CUL3 genes lead to increased expression of NRF2 and its target genes in lung cancer. Thus, these studies provide ample evidence for the dual role of NRF2 in lung cancer. In this review, we discussed the mechanistic insights into the role of NRF2 signaling in therapy resistance by focusing on cell lines, mouse models, and translational studies in lung cancer. Finally, we highlighted the potential therapeutic strategies targeting NRF2 inhibition, followed by the discussion of biomarkers related to NRF2 activity in lung cancer. Overall, our article exclusively discusses in detail the NRF2 signaling pathway in resistance to therapy, especially immunotherapy, and its therapeutic avenue in the treatment of lung cancer.