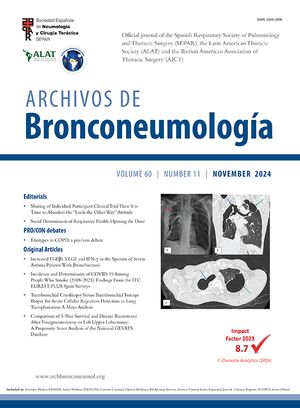
Immune checkpoint inhibitors have opened an era of lung cancer therapy. However, a notable disparity exists in the efficacy of immunotherapy among individual patients. The tertiary lymphoid structure (TLS) is an ectopic lymphocyte aggregation that appears under pathological conditions and is the primary site of action for anti-tumor immunity. It is commonly reported that the presence of TLS within the tumor microenvironment (TME) relates to a favorable clinical prognosis and an excellent response to immunotherapy in lung cancer patients. A thorough understanding of TLS and its dynamic changes in TME has become an attractive focus for optimizing immunotherapy strategies for lung cancer. In this review, we comprehensively generalize the composition, formation, mechanism, detection methods of TLS, and summarize the role of TLS in lung cancer immunotherapy. Finally, induction of TLS is also discussed, which may provide more effective therapeutic strategies for lung cancer therapy.